What are we studying? | Why are we studying? | What are we finding? | What does it mean? | Where can I read more?
What are we studying?
We are studying engineered nanoparticles and their potential to cause cancer. Nanoparticle exposure is increasing as nanoparticle use rises worldwide due to their extensive use in consumer products. Our work is focused on determining the effects of nanoparticles on DNA and genomic stability, and their potential to cause cancer. We are using human lung cells, to determine the cellular and molecular effects of metal nanoparticles including gold, silver, and copper. We are considering the possible effects of size, agglomerate properties, composition, shape and surface composition, and determining how these parameters affect the ability of metal nanoparticles to damage DNA (measured as strand breaks and chromosome damage) and induce genomic instability.
Why are we studying it?
Nanotechnology is considered to be the next industrial revolution and likely to soon become a $1 trillion dollar industry within the next 10 years Billions are already being invested in nanotechnology. Nanoparticles are used in a wide array of commercial products including sunscreens, clothing, semiconductors, tires, drug delivery systems and more. We are in the beginning of the coming age of nanotechnology.
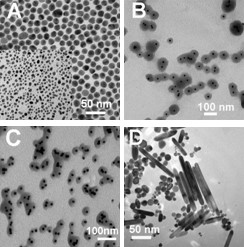
Nanoparticles are defined as having at least one dimension with a size less than 100 nm. Their properties are determined on the quantum scale, which means their properties are defined by quantum mechanics. It also means which they don’t follow the known laws of solids, liquids or gases that larger particles do. As a result, nanoparticle have mechanical, magnetic, electronic, color and other properties that are different then these same chemicals would have on at larger size scales. For example, gold particles were considered to be almost completely inert, and as such have historically not been investigated for commercial uses as a chemical reactant. However, on the nano scale gold nanoparticles can have highly active properties making them strong catalysts. Consequently, a whole new industry is emerging with vastly significant new products and markets.
However, the same properties that make these particles exciting in technology and consumer markets also make them daunting public health concerns. Simply put, whether or how these new properties will enhance, diminish or otherwise alter the toxicity of the compounds from which they are derived is not known. The toxicity of nanoparticles is unknown and relatively unexplored. This lack of data is remarkable given the use of the technology in high consumer-use products like clothes and sunscreen. To help understand the health risk of exposure to these materials, we are studying the toxic effects of nanoparticles.
What are we finding?
We have published data of the impact of silver nanoparticles in fish cells. We have found silver nanoparticles can cause cell death and damage chromosomes in these cell. By contrast, we found titanium nanoparticles did not cause cell death or damage chromosomes in human cells.
What does it mean?
Our data support the suggestion that nanoparticles may poses a potential health risk to people who are exposed to it through either inhalation of nanoparticles or other routes such as diet or drinking water. But, care must be taken as there will be significant differences in the types of nanoparticles.
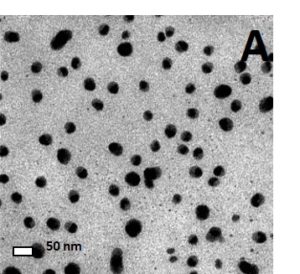
Particle characterization is an important step in assessing the toxicological effects of nanoparticles as particle synthesis, particle size, and particle concentration and agglomeration can all affect the toxicity of nanoparticles. Using both transmission electron microscopy (Figure 2) and dynamic light scattering (Figure 3(2)), we have determined that the mean size of our silver nanoparticles are ~30 nm (18). The particle diameter results obtained by dynamic light scattering yielded three peaks (Figure 3). The peak at 8 nm is most likely due to abundant nano-sized particulates present in the cell culture media and does not represent silver nanoparticles. The second peak represents the diameter of single silver nanoparticles with a mean diameter of 32 nm which is consistent with the TEM data. The third peak represents agglomeration of the silver nanoparticles which occurs in the presence of electrolyte ions or due to an equilibrium shift in citrate ions after the particles are diluted in culture media. As shown in figure 4, agglomeration increases as concentrations increases and agglomeration is not due the presence of cells or serum in the media. In fact the proteins in the serum decrease agglomeration.
DNA Damage and Repair Studies
In addition to investigating the effects of nanoparticles on human cells, we have also begun to investigate their effects on fish cells. Nanoparticles, specifically silver nanoparticles, are likely to contaminant the aquatic environment due to their use in washing machines and clothes and it is important to elucidate their effects on cells from model aquatic organisms. For these studies, we used adult fin cells froma small, teleost fish, the medaka (18). We found that exposure to silver nanoparticles for 24 h induced chromosome damage (Figure 5) in the form of chromatid lesions (Figure 6A), isochromatid lesions (Figure 6B), chromatid exchanges (Figure 6C) and centromere spreading (Figure 6D) (18). The presence of these lesions suggests that silver nanoparticles can induce structural chromosome instability. Structural chromosome instability is a hallmark of cancer cells aschanges in chromosome structure can lead to deletions of genes or rearrangements that increase the levels of some proteins and decrease others. Current efforts are aimed at investigating whether silver nanoparticles can induce structural chromosome instability in human cells and whether DNA double strand breaks are induced after silver nanoparticle exposure.
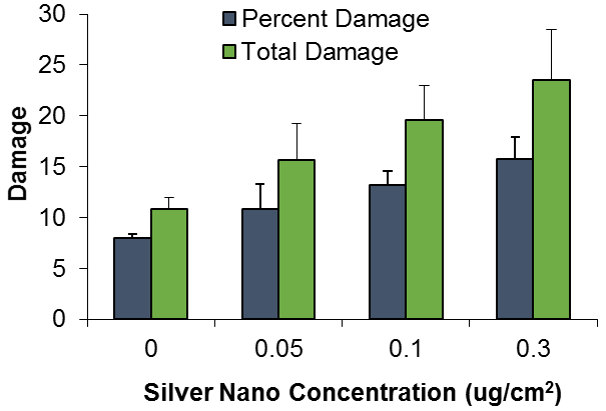
Mitosis Studies
Another form of chromosome instability is numerical chromosome instability. We found that silver nanoparticles induced numerical chromosome instability in medaka cells (Figure 7) (18). Multiple mechanisms exist that give rise to numerical chromosome instability, including centrosome amplification and spindle assembly checkpoint bypass. Future efforts are aimed at investigating the mechanism of silver nanoparticle-induced numerical chromosome instability.
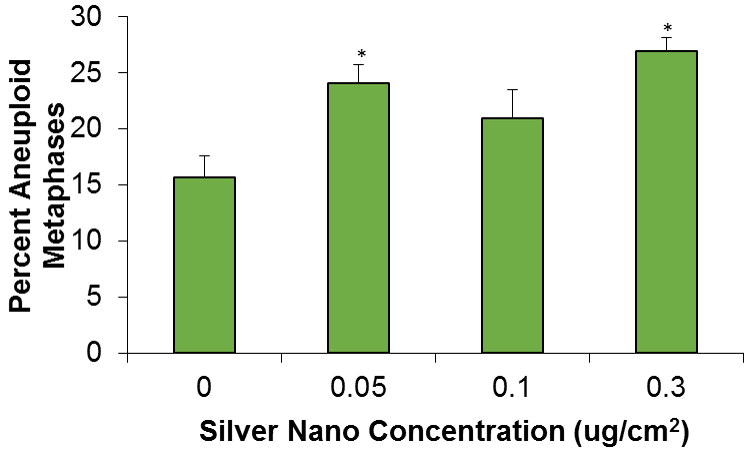
Where can I read more?
- Wise, Sr., J.P., Goodale, B.C., Wise, S.S., Craig, G., Pongan A.F., Walter, R.B., Thompson, W.D., Ng, A.K., Aboueissa, A.M., Mitani, H., Spalding, M.J. and Mason, M.D. Silver Nanospheres Are Cytotoxic and Genotoxic to Fish Cells. Aquatic Toxicology, 97: 34–41, 2010. PMID: 20060603. PMCID: PMC4526150.
- Xie, H., Mason, M.M. and Wise, Sr., J.P. Genotoxicity of Metal Nanoparticles. Reviews on Environmental Health, 26(4): 251-268, 2011. PMID: 22435324.
- Browning, C., The, T., Mason, M.D. and Wise, Sr., J.P. Titanium Dioxide Nanoparticles Are Not Cytotoxic or Clastogenic in Human Skin Cells. Journal of Environmental and Analytical Toxicology, 4-6: 1-6, 2014. http://dx.doi.org/10.4172/2161-0525.1000239. PMID: 26568896. PMCID: PMC4643289.